Scientific Rationale for the Use of Metabolic Activators for Treatment of Metabolic Diseases
Summary. Mitochondria are energy factories of cells and are important pivots for intracellular interactions with other organelles in the human cells. Based on artificial intelligence and systems biology analysis, it has been found that a mixture of metabolic activators can be used in activating the mitochondria and improving metabolic health in patients with metabolic abnormalities. The approach combines systems biology and clinical medicine in a manner not previously done. A connection between low levels of NAD+ and antioxidants (glutathione), abnormal metabolic activities and declined mitochondrial activity has been established with metabolic disorders e.g. Non-Alcohol Fat Liver Disease (NAFLD), COVID-19, Gout and certain neurological diseases. These results open the door to entirely novel treatments for patients by restoring levels of Nicotinamide adenine dinucleotide (NAD+) and antioxidant providing entirely novel strategies for the treatment of the patients with diseases with metabolic and mitochondrial involvement.
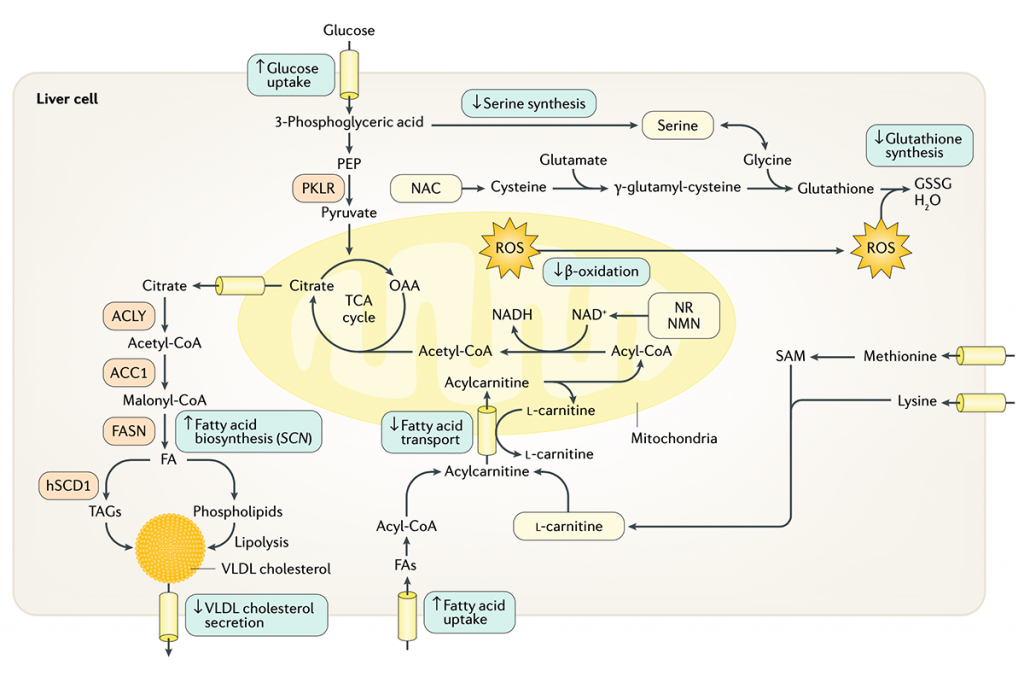
.
Figure 1. A schematic view of energy metabolism in the cell. Natural substances, including serine, glycine and N- acetylcysteine (NAC), could be supplemented to boost the level of glutathione; the precursors of NAD+, including nicotinamide riboside (NR)/nicotinamide mononucleotide (NMN) and Niacinamide, could be supplemented to boost the level of NAD+; and L -carnitine could be supplemented to boost the transfer of FAs to the mitochondria. Supplementation of such natural substances (shown in yellow boxes) might eventually promote mitochondrial FA oxidation in the liver, which can decrease the level of the accumulated fat in the liver. GSSG, glutathione (oxidized); OAA , oxaloacetate; PEP, phosphoenolpyruvate; ROS, reactive oxygen species; TAG, triacylglycerol; TCA , tricarboxylic acid; SAM, S- adenosyl-L- methionine.
.
.
The first genome-scale model of metabolic disorders. In 2014, we constructed a liver-specific Genome-Scale Metabolic Model (GEM) following the generation of liver transcriptomics data, and use this computer model to study the pathophysiological responses of hepatic disorders (1). This consensus functional GEM for hepatocytes, (iHepatocytes2322) was generated by integrating existing biochemical knowledge with the proteomics and transcriptomics data generated in the Human Protein Atlas (HPA) project (2). The proteomics and transcriptomics data have been generated using the liver biopsy samples from NAFLD patients and healthy subjects. This computer model has been used to analyse the transcriptomics data generated for different stages of NAFLD using Artificial Intelligence and Systems Biology approach. Our analysis provided new insights into the metabolic reprogramming that occurs in connection with Non-alcoholic Steatohepatitis (NASH) development. Integration of the transcriptomics and proteomics data led to identification of potential biomarkers (chondroitin and heparan sulfates) for diagnosing NASH and for the staging of NAFLD.
An AI-based model for treating metabolic disorders. Moreover, the study indicated that patients with NASH display serine deficiency, suggesting that increasing serine levels by dietary supplementation will be beneficial for these patients. These findings were also in agreement with metabolomics data that showed lower plasma levels of serine in patients with NAFLD than in healthy individuals (1). This AI-based model was also used for the evaluation of possible novel treatment strategies by targeting one or more of the metabolic processes that cause NAFLD. The objective was to increase endogenous fatty acid synthesis, improve delivery of fatty acids to the liver and to decrease mitochondrial fatty acid oxidation. In addition, the aim is to treat the imbalance in the export of triglycerides as VLDL cholesterol.
Flux data to simulate liver metabolism. In 2017, results from stable-isotope studies of triglyceride-rich VLDL cholesterol kinetics were combined with experimentally derived flux data to simulate liver metabolism using iHepatocytes2322 (4). The intracellular fluxes in the liver tissue of each of 86 patients involved in the study were predicted, and the correlations between the intracellular fluxes of the liver in patients with increased hepatic steatosis were assessed to elucidate the molecular derangements in the pathogenesis of NAFLD. The integrated systems approach indicated that altered NAD+ and glutathione metabolism (with an increased demand for NAD+ and glutathione) was a prevailing feature in NAFLD. Integrative analysis and plasma metabolomic data further indicated that plasma levels of serine, glycine and precursors of glycine are significantly negatively correlated with hepatic steatosis and suggested that serine/glycine is the limiting substrate for de novo glutathione synthesis.
First human studies in patients with liver disease. To confirm these results and those of the earlier study indicating serine deficiency in patients with NAFLD, a short-term dietary supplementation study with one oral dose of ~20 g of L -serine (200 mg/kg body weight) per day was performed for 14 days in six patients with extensive hepatic steatosis (4). Serine supplementation was well tolerated by all the patients, and plasma levels of the liver enzymes alanine aminotransferase, aspartate transaminase and alkaline phosphatase — all well-known markers for liver health — significantly decreased after supplementation. Moreover, using magnetic resonance spectroscopy, it was found that hepatic steatosis significantly decreased after serine supplementation. Furthermore, a system-level analysis suggested that a three-step strategy, including increased fatty acid uptake by mitochondria, fatty acid oxidation and generation of glutathione, might be advantageous for patients with NAFLD. Supplementation of the diet with natural substances including L -carnitine can activate the fatty acid uptake, precursors of NAD+, including nicotinamide riboside or nicotinamide mononucleotide, can activate the fatty acid oxidation, and serine, glycine or N-acetylcysteine can activate the generation of glutathione, which might lead to a decrease in the level of the fat accumulated in the liver.
Human intervention studies. In 2018, we performed a short-term intervention with an isocaloric low-carbohydrate diet with increased protein content in obese subjects with NAFLD and characterized the resulting alterations in metabolism and the gut microbiota using a multi-omics approach (5). We observed rapid and dramatic reductions of liver fat and other cardiometabolic risk factors paralleled by (i) marked decreases in hepatic de novo lipogenesis; (ii) large increases in serum b-hydroxybutyrate concentrations, reflecting increased mitochondrial b-oxidation; and (iii) rapid increases in folate-producing bacteria and serum folate and serine concentrations. Liver transcriptomic analysis on biopsy samples from a second cohort revealed downregulation of the fatty acid synthesis pathway and upregulation of serine/folate-mediated one carbon metabolism and fatty acid oxidation pathways.
Human calibration study of a combined metabolic activator formulation. In 2019, we first assessed the tolerability of the combined metabolic activators including L-serine, N-acetyl-L-cysteine (NAC), nicotinamide riboside (NR), and L-carnitine by performing a 7-day rat toxicology study. Second, we performed a human calibration study by supplementing combined metabolic activators (CMAs) and a control study to study the kinetics of these metabolites in the plasma of healthy subjects with and without supplementation. We measured clinical parameters and observed no immediate side effects. Next, we generated plasma metabolomics and inflammatory protein markers data to reveal the acute changes associated with the supplementation of the CMAs. We also integrated metabolomics data using personalized genome-scale metabolic modelling and observed that such supplementation significantly affects the global human lipid, amino acid, and antioxidant metabolism. Finally, we predicted blood concentrations of these compounds during daily long-term supplementation by generating an ordinary differential equation model and liver concentrations of serine by generating a pharmacokinetic model and finally adjusted the doses of individual metabolic activators for future human clinical trials. In animal experiments and human kinetic study, we found that CMAs promotes the oxidation of fat, attenuates the resulting oxidative stress, activates mitochondria and eventually removes excess fat from the liver.
Human phase 2 in fat liver patients. In 2020, we completed a phase 2 clinical trial testing the safety and efficacy of the combined metabolic activator (CMA) formulation in NAFLD patients in a placebo-controlled 10-week study (6). We found that CMAs significantly decreased hepatic steatosis and levels of aspartate aminotransferase, alanine aminotransferase, uric acid, and creatinine whereas found no differences on these variables in the placebo group after adjustment for weight loss. By integrating clinical data with plasma metabolomics and inflammatory proteomics as well as oral and gut metagenomics data, we revealed the underlying molecular mechanisms associated with the reduced hepatic fat and inflammation in NAFLD patients and identified the key players involved in the host-microbiome interactions. In conclusion, we observed that CMAs could develop a pharmacological treatment strategy in NAFLD patients.
Human phase 3 clinical trials for COVID-19 patients. COVID-19 is associated with metabolic abnormalities, including the deficiencies in nicotinamide adenine dinucleotide (NAD+) and glutathione metabolism. In 2020, we also conducted randomised, placebo-controlled an open-label phase 2 study and a double-blinded phase 3 clinical trials for testing if a mixture of Combined Metabolic Activators (CMA) consisting of NAD+ and glutathione precursors had beneficial effects on ambulatory COVID-19 patients (7). We randomly assigned 93 and 304 patients in phase 2 and phase 3, respectively, and administered CMA or placebo for 14 days. We evaluated the clinical status daily using a binomial scale for presence/absence for COVID-19 symptoms. The results of both studies showed that the time to complete recovery was significantly shorter in the CMA group (6.6 vs 9.3 days) in phase 2 and (5.7 vs 9.2 days) in phase 3 trials independent of standard therapy. We unveiled the molecular changes by generating untargeted metabolomics and inflammatory proteomics data for all patients recruited in the phase 2 trial, showing that the plasma levels of proteins and metabolites associated with inflammation and antioxidant metabolism are significantly improved. The results suggests that combination therapy is safe and beneficial in patients with mild-to-moderate COVID-19.
The scientific rationale – a summary. The aim of the novel drug treatment based on combined metabolic activators is to increase the level of NAD+ and glutathione in cells and tissues to boost the function of the mitochondria. We have used natural substances, including serine, glycine and/or N- acetylcysteine (NAC) to increase the level of glutathione. In addition, precursors of NAD+, suitable for oral administration, such as nicotinamide riboside (NR) and nicotinamide mononucleotide (NMN), are added to the formulation to increase the level of NAD+ in cells and tissues. Finally, the natural transport molecule L-carnitine is added to increase the transfer of fatty acids into the mitochondria of the cell. An effect of the activation of the mitochondria is also to decrease the level of the accumulated fat in the liver. All of these natural substances can be administrated orally to promote mitochondrial fatty acid oxidation and thus potentially help patients with mitochondrial dysfunction.
References
- Mardinoglu, A. et al. Genome- scale metabolic modelling of hepatocytes reveals serine deficiency in patients with non- alcoholic fatty liver disease. Nat. Commun. 5, 3083 (2014).
- Uhlen, M. et al. Tissue- based map of the human proteome. Science 347, 1260419 (2015).
- Kalhan, S. C. et al. Plasma metabolomic profile in nonalcoholic fatty liver disease. Metabolism 60, 404–413 (2011).
- Mardinoglu, A. et al. Personal model- assisted identification of NAD+ and glutathione metabolism as intervention target in NAFLD. Mol. Syst. Biol. 13, 916 (2017).
- Mardinoglu et al., An Integrated Understanding of the Rapid Metabolic Benefits of a Carbohydrate-Restricted Diet on Hepatic Steatosis in Humans, Cell Metab. (2018),
- Zeybel et al., Combined Metabolic Cofactor Supplementation Reduces Liver Fat in Nonalcoholic Fatty Liver Disease, Under review at Cell Metabolism.
- Altay et al., Combined Metabolic Activators accelerates recovery in mild-to-moderate COVID-19, Under review at Advanced Science